Quantum computing harnesses the principles of quantum mechanics to process data in a fundamentally different way compared to traditional computers. Instead of using bits (0s and 1s), a quantum computer employs quantum bits, or qubits, which can represent both 0 and 1 simultaneously. This is made possible by a physics property known as superposition.
Imagine a coin. It has two sides: heads and tails. In traditional computing, the coin can either be heads up or tails up on the table. In quantum computing, a qubit is like a spinning coin, representing both at once. This capability allows for complex calculations at much faster rates, as it can process a vast array of possibilities simultaneously. Still confused?
Consider planning a cross-country road trip. You have multiple routes to choose from. A traditional computer would calculate the time for each route sequentially to give you the shortest or quickest one.
A quantum computer, however, could evaluate all routes simultaneously, quickly pinpointing the best option for you. The power of quantum computing lies in its ability to process numerous possibilities at once.
The Impact of Quantum Computing on Business Industries
What role does quantum computing play in business? Quite a significant one, actually. Data processing is currently limited by how quickly it can be calculated. Quantum computing can solve problems more efficiently than traditional computers, potentially transforming industries heavily reliant on data.
Take finance, for instance, which depends on vast amounts of data. Quantum computing could enable companies to perform risk assessments and detect fraud by analyzing large data sets instantaneously. This would also lead to more accurate predictions and forecasts.
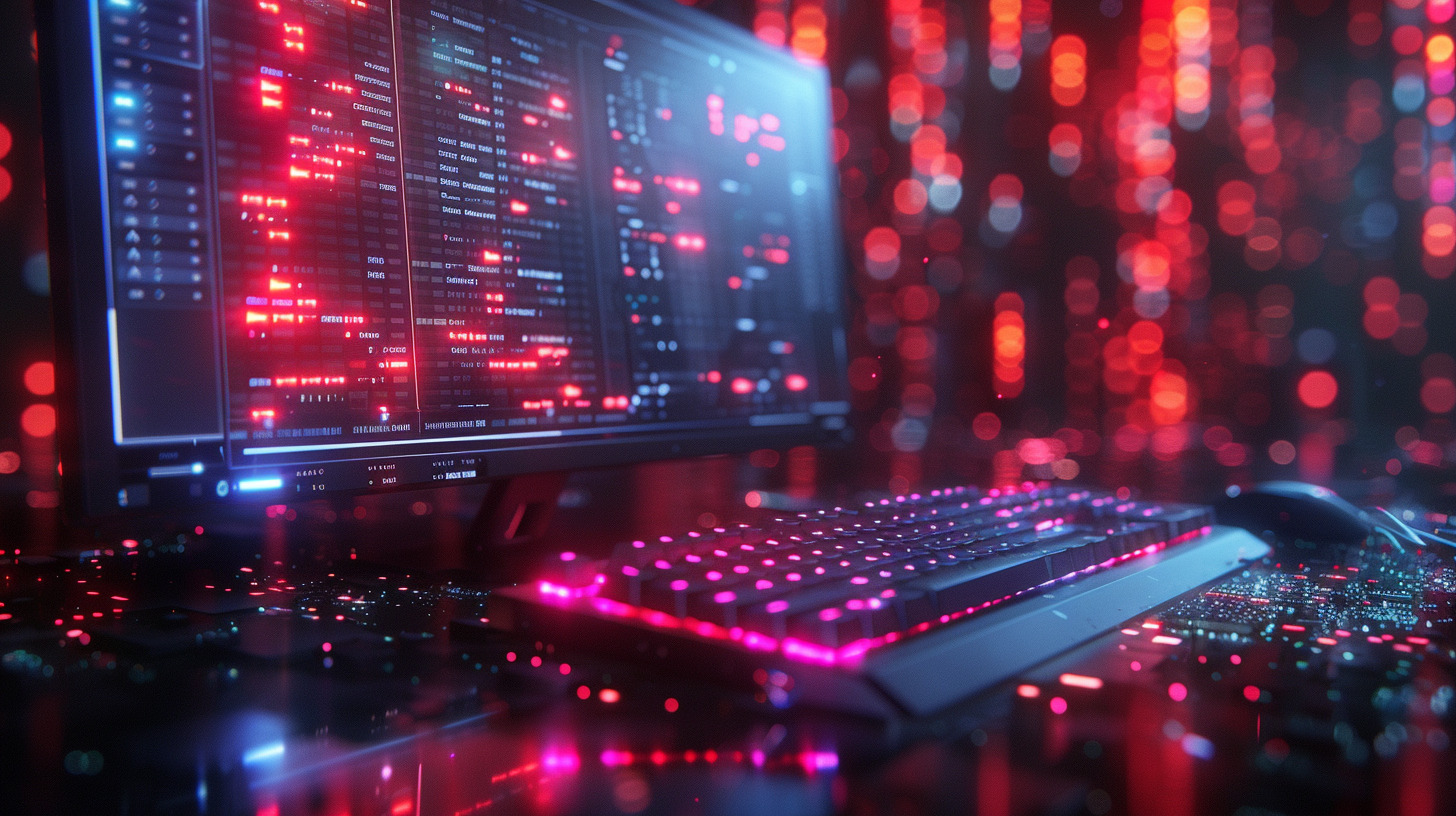
In pharmaceuticals, quantum computing can simulate molecular structures, potentially streamlining the drug discovery process and reducing the time and cost involved in bringing new treatments to market.
Princeton’s Advances in Quantum Error Correction
Recently, an engineer who leads quantum initiatives at a prestigious university shared that his and his colleagues’ attention is shifting from the smaller models of the NISQ era to much larger ones. This transition is driven by the industry’s recognition that large and robust systems are essential for realizing the practical benefits of quantum computing.
Expanding on this idea, they discussed the challenges and innovations in quantum computing, particularly in quantum error correction. While theoretical frameworks for error correction have been well-established, implementing them in practice remains challenging. The key question is how to move these theories from the lab to real-world applications.
Detecting and correcting errors efficiently in real-time is vital for the operation of large quantum systems. Robust error correction mechanisms are crucial for developing reliable, scalable quantum computers.
A Gradual Transformation
In my view, quantum computing will be transformative, but more through incremental advancements than abrupt changes. We are likely to witness a gradual integration of quantum computing into existing systems, allowing industries to adapt and evolve with the new technology over time.
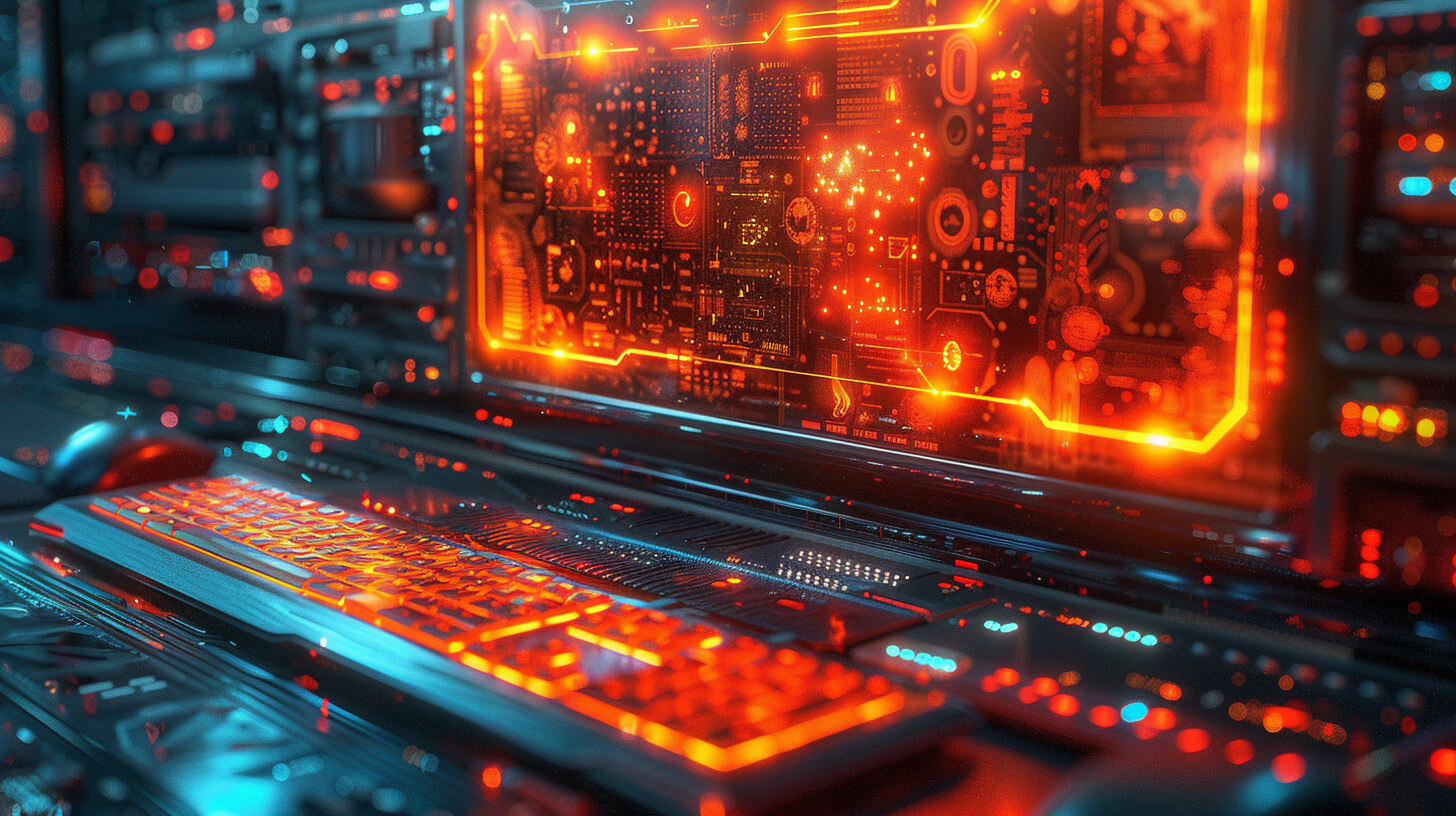
History provides insight through the invention of the transistor. Created in 1947 by John Bardeen, Walter Brattain, and William Shockley at Bell Labs, the transistor gradually revolutionized technology through a series of smaller developments that unveiled its vast potential.
Initially considered a technical curiosity, the transistor eventually replaced vacuum tubes in radios, computers, and numerous other electronic devices. Its introduction marked the dawn of a new era in electronics, leading to the development of smaller, more reliable, and energy-efficient devices.
This shift did not occur overnight; it was the result of persistent experimentation, refinement, and integration into existing technologies. Just as the transistor enabled the miniaturization and enhancement of countless electronic devices, quantum computing is poised to unlock new capabilities in computing power, processing speed, and problem-solving efficiency.
Though its full potential might not be realized immediately, its gradual integration across various fields promises to be as transformative, if not more so, than the shift from vacuum tubes to transistors.